1. Introduction
Bone is a tissue that is consist of organic and inorganic materials with a micro/nanocomposite structure. The bone matrix is composed of collagen as the organic phase (about 35% of dry weight), carbonated apatite as the mineral phase (about 65% of dry weight), and other non-collagenous proteins (stimulating microenvironment for cellular functions) [
1,
2,
3]. The collagen causes bone hardness, viscoelasticity, and toughness, whereas the inorganic phase handles the structural strengthening and firmness [
1]. The combination and construction of the bone, including mineralization, the configuration of collagen fibers, porosity, and trabecular vs cortical bone architecture determines its mechanical properties [
1,
4].
Problems related to bones are among the illnesses and abnormalities that have become one of the most important and serious troubles that should be addressed. Significant bone defects can occur for a variety of reasons, including fractures because of traumatic osteoporosis and skeletal defects resulting from the removal of primary and metastatic tumors, post-skeletal bone resorption, complete joint arthroplasty, osteoarthritis of the spine, aging, and congenital malformations; as a result, people’s daily lives are severely hampered and their quality of life is reduced [
5,
6,
7,
8,
9,
10]. Although the bone tissue incorporates a considerable capacity to recover and repair itself, it is not possible to completely and continuously repair itself if the bone defects become more than the crucial size. Consequently, their treatment requires implementing a proper bone graft at the defect site which is a major challenge. Hence, bone tissue replacements in surgeries, such as autografts, allografts, and xenografts are essential to treat such defects [
11]. Considering the problems with using autografts, allografts, and xenografts, patients tend to use engineered materials that can imitate the properties of the natural bone, thereby avoiding further issues. Therefore, artificial materials for bone repair have been considered by scientists and researchers. A variety of materials are created individually or compositely to meet clinical needs [
5,
12,
13,
14,
15,
16]. For this reason, the creation of various types of synthetic materials has attracted scientists’ and researchers’ attention to resolve clinical needs. The following are among the considered materials as bone cement for bone tissue engineering: various composites (types of organic/inorganic composite systems); bioactive glass; absorbable and inert ceramics, such as calcium phosphate, calcium sulfates, coating; and scaffolds. Also, polymers (biodegradable/non-biodegradable) and metals have been extensively studied as potential materials for repair [
17,
18,
19,
20].
Bone cement is a self-curing chemical compound (synthetic organic/inorganic material) that has been used successfully in orthopedic and osteotomy surgeries to strengthen and stabilize weak or damaged bones using artificial joints (prostheses). In such operations, the defective joint or bone is removed and replaced with a new limb. In this way, when replacing a bone or joint, they create holes in which the cement is poured. The cement hardens after a while like glue and causes the bones to completely weld together (
Figure 1) [
21,
22,
23,
24,
25,
26,
27,
28].
.jpg)
The exchange of bone constrain to the implant and the implant to the bone is the main function of bone cement. The cement can reduce the workforce evenly on the bone because of its desirable stiffness. Considering its long-term durability, easy shaping, and self-adjusting properties, cement is a strong and reliable anchor material. In addition, given the negligible auxiliary harm, reduction of hospitalization time, and ease of use in clinical practice, millions of joint replacements are performed annually worldwide, more than half of which use bone cement, and this trend is increasing [
29,
30,
31].
Types of bone cement
Acrylic or Polymethyl Methacrylate (PMMA) based cement, Polypropylformite (PPF) based cement, Calcium Sulfate Cement (CSC) (Paris gypsum), Calcium Phosphate Cement (CPC), magnesium phosphate cement, Calcium Silicate Cement (CSiC), Calcium Aluminate Cement (CACs), and glass ionomer cement are among the currently used materials in the clinic [
32,
33,
34,
35,
36,
37,
38,
39].
Acrylic cement or polymethyl methacrylate based cement
Acrylic or PMMA-based cement is the oldest and most famous group of bone cement [
40] and has various applications in dentistry and optometry. PMMA microspheres have been used to strengthen soft tissues for body fat atrophy and facelift [
41]. It is also widely used in orthopedics to fix prostheses to bones. PMMA has many advantages, including biological ineffectiveness, ease of operation, significant mechanical strength, and affordability; hence, it is considered an ideal bone cement [
41,
42].
Polypropylformite based cement
PPF is one of the synthetic polymers used in bone tissue engineering for pre-clinical applications. Therefore, the applications of PPF-based materials in cardiac tissue engineering, ophthalmology, drug delivery (
Figure S1), and neural tissue engineering have been investigated.
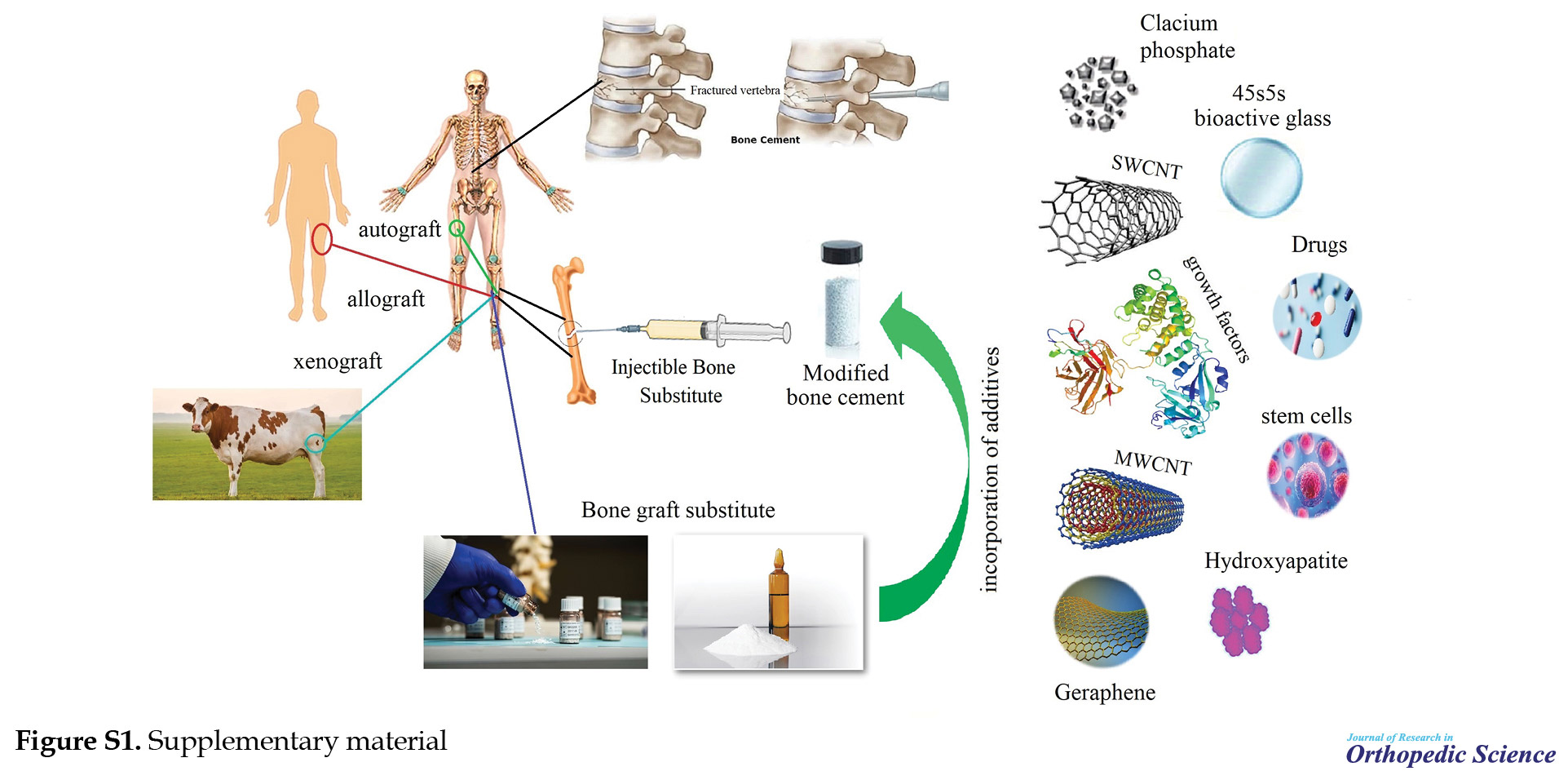
In recent years, advances in synthetic methods, scaffolding, and agent fabrication, especially in 3D printing techniques (e.g., stereolithography [SLA] and continuous digital light processing [cDLP]) have created new opportunities in the use of PPF-based materials (bone regeneration and drug delivery) (
Figure 2) [
10].
Calcium sulfate cement
CSC (plaster of Paris) is an alternative (superseded) material for use in bone grafting in different places. CSC has a long clinical history. It can be used in the transportation of drugs, and growth factors, or as a soluble additive to adjust the porosity or the rate of biodegradation when combined with other biological materials [
43,
44].
Calcium aluminate cement
In the late 1990s, the use of CAC as an alternative to amalgam for tooth restoration was introduced by the Swedish company Doxa Certex AB. Recently, its applications in orthopedics have also developed [
38].
Calcium silicate cement
CSiCs are among the injectable materials that can be used in endodontic treatment. Mineral trioxide aggregate (MTA) consists of tricalcium silicate, dicalcium silicate, Tricalcium Aluminate (TCA), tetra calcium aluminophyte, and calcium sulfate, along with bismuth oxide as the radiopacifying agent. The paste created as a result of the combination with water can be molded at the implant site [
37].
Calcium phosphate cement
CPBCs are compounds used in the body during the osteogenesis process. Scaffolds made using calcium phosphate can be applied in dentistry and orthopedics [19, 45]. The first calcium phosphate bone cement was invented by Brown and Chow in 1987. The clinical potentials of calcium phosphate material further increased because of its properties, such as low curing temperature, proper degradability, passive/active assimilation, the capability to turn into bone within months to years, non-toxic end products, intrinsic microporous structure (their porosity is approximately 30-50), non-shrinkage of the cement during the hardening process, and injectability [
34,
37,
38,
42,
45,
46,
47,
48]. In addition, CPBCs are considered a clinical alternative to traditional bioceramics and potentially serve as an ideal filler for vertebroplasty and balloon kyphoplasty applications, as well as in oral and maxillofacial surgeries, and the implantation of periodontal implants and pulp and mineral polish of teeth [
34,
41,
49,
50]. So far, hundreds of CPBCs, with different formulations have been developed to create new and injectable biomaterials [
5].
Magnesium phosphate cement
MPC is another group of bioactive and degradable injectable bone cement. Yang et al. mixed CaS powder with magnesium phosphate (MgP) to form CaP/MgP bone cement [
51].
Glass ionomer cement
Glass ionomer cement is one of the materials used in dentistry [
52,
53,
54]. This cement was introduced according to the standard of the International Organization for Standardization as a glass poly-alkenoate cement [
55]. Glass ionomers were invented in the late 1960s by Wilson and colleagues at the LGC Laboratory in London to replace silicate cement [
56]. The first cement with good properties was known as G200, and it was produced from this glass; it was known as ASPA I [
57]. ASPA II cement was introduced by making changes in the glass formulation by adding tartaric acid. Glass ionomer cement has evolved over the past few decades and various components have been added to the system as reinforcements [
58].
Glass carbomer
Glass Carbomer is a new commercial ionomer glass material whose biocompatibility has increased compared to conventional ionomer glass cement. This material is made by GCP Dental in the Netherlands [
59].
Chemical composition and chemistry of bone cement
Bone cement is a self-curing system that is available on the market as two-component materials (liquid phase in a dark ampoule and solid phase as a white powder in a sterilized pack. The solid phase is then mixed in the operating room) (
Figure 3) [32].
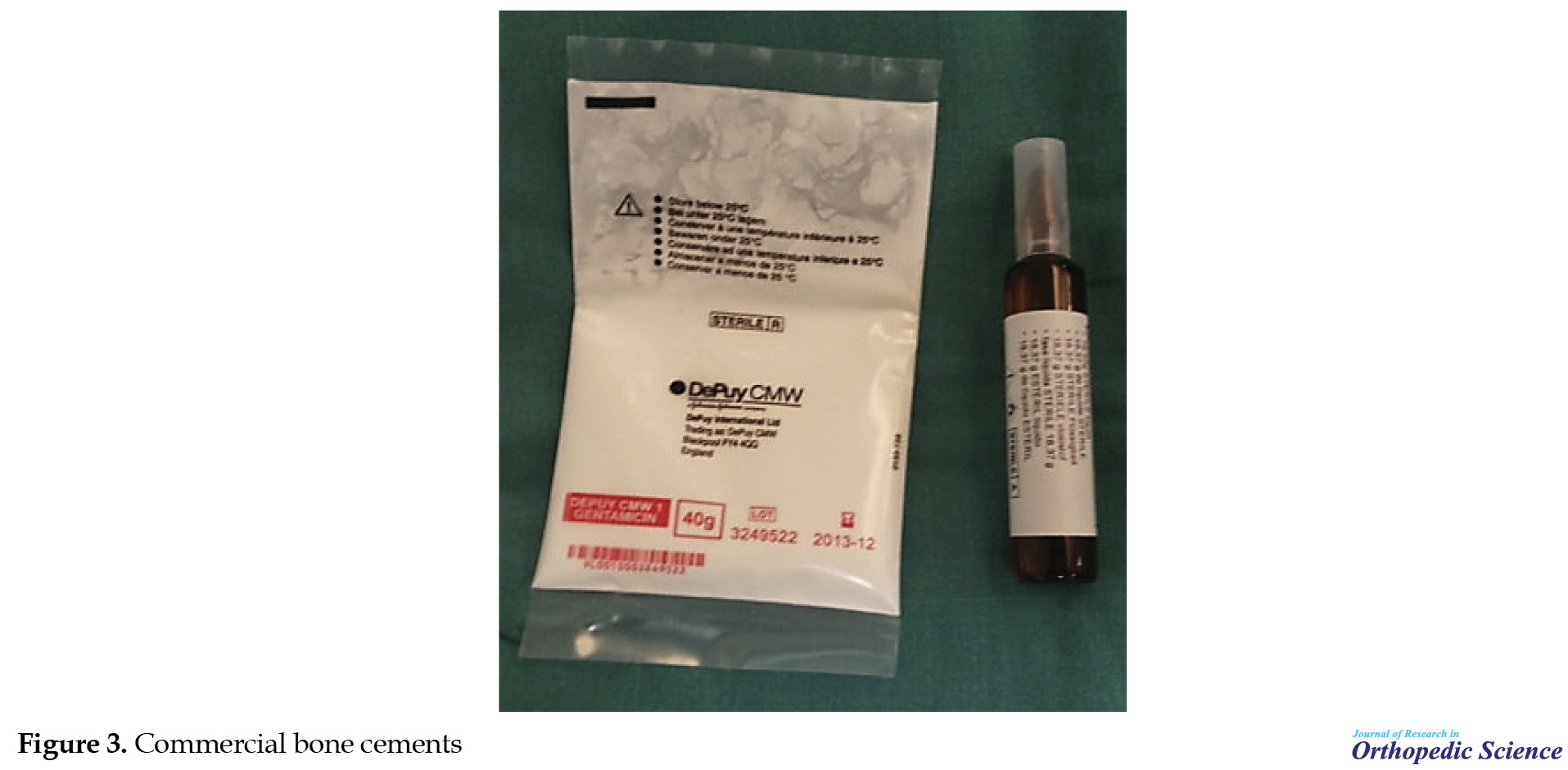
Different types of bone cement have been developed and are commercially available [
24,
31,
60,
61,
62]. These formulas have been optimized over the years to provide the best in vivo performance [
40]. The components of each cement are as follows:
Acrylic or Polymethyl Methacrylate: The solid phase consists of granules (spherical particles) of poly (methyl methacrylate) prepolymer (PMMA) and/or Acrylic Acid (AA) copolymers (ethyl acrylate [EA]); Methyl Acrylate (MA); MMA and styrene initiator benzoyl peroxide (BPO); or Tri-N-Butyl Borane (TBB) (in the commercial product Bonemite); radiopaque agent (radiopacifiers) as X-ray contrast agents containing mineral compounds, such as soul powders barium, zirconium dioxide, tantalum, and tungsten. The liquid phase (liquid part, liquid component) consists of MMA monomer (butyl methacrylate), N-decyl methacrylate (DMA) or isobornyl methacrylate (IBMA) as comonomers, accelerator (activator) mainly N, N-dimethyl -p-toluidine (DMT) or 2- (4-dimethlylamino) phenyl ethanol as a hydroquinone inhibitor or di-tert-butyl-p-cresol and sometimes a cross-linking agent as Ethylene Glycol Dimethacrylate (EDGMA) [
31,
37,
40,
41,
42,
46,
49,
63,
64].
Occasionally, dyes or pigments, such as chlorophyll or methylene blue are added to stain bone cement so that bone cement can be easily identified after surgery [
46,
65].
Calcium Phosphate Cement: The solid phase comprises calcium phosphate (one or more compounds) (CaP) (e.g., calcium phosphate dihydrate + tetra calcium phosphate (CPD+TTCP), monocalcium phosphate monohydrate + β-tricalcium phosphate [MPCM + β-TCP], and α-tricalcium phosphate + monocalcium phosphate monohydrate + calcium oxide + hydroxyapatite [α-TCP + MPCM + CaO + HA]). The radiopaque agent as an X-ray contrast agent contains mineral compounds, such as barium sulfate powders, zirconium dioxide, tantalum, and tungsten.
The liquid phase consists of water or an aqueous solution, containing calcium or phosphate, dilute phosphoric acid, saline, or immiscible liquids with water or ovine whole blood [
5,
42,
46].
Calcium Sulfate Cement: The powder phase is calcium sulfate hemihydrate, because of the combination of the powder phase of calcium sulfate hemihydrate with a diluent (water), calcium sulfate dihydrate is obtained. It has a paste or putty with a solid or semi-solid structure and hardens after approximately 5 min. MIIG X3 is one of the most popular and widely used available brands [
39].
CaSO4.0.5H2O +1.5 H2O → CaSO4.2H2O
Glass Ionomer Cement: Glass ionomer cement is composed of silica, alumina, calcium fluoride, a polymeric acid (containing the carboxylate group), water, and some complexing agent (tartaric acid) [
55,
66,
67,
68].
Glass Carbomer Cement: Glass carbomer cement’s components are glass powder and silicone oil containing polydimethylsiloxane, whose structure is linear and includes hydroxyl groups [
59].
Preparation of bone cement
As mentioned previously, the most available bone cement on the market consists of two parts: a package that contains PMMA powder and an ampoule that contains monomeric MMA liquid. To prepare the cement, solid and liquid phases are mixed in a container, usually at room temperature. For this purpose, the bag containing the powder is cut with sterile scissors and its contents are transferred to a container. Then, the contents of the ampoule are added and mixed until a homogeneous paste is obtained (1 to 3 min). Subsequently, a vacuum and or centrifuge is applied to the mixture until the gas bubbles are created during mixing and polymerization are removed. Also, by reducing the porosity of the cement, the quality of the cement is improved. Finally, the dough is put in the specified area by the surgeon (
Figure 4A-C)[49].
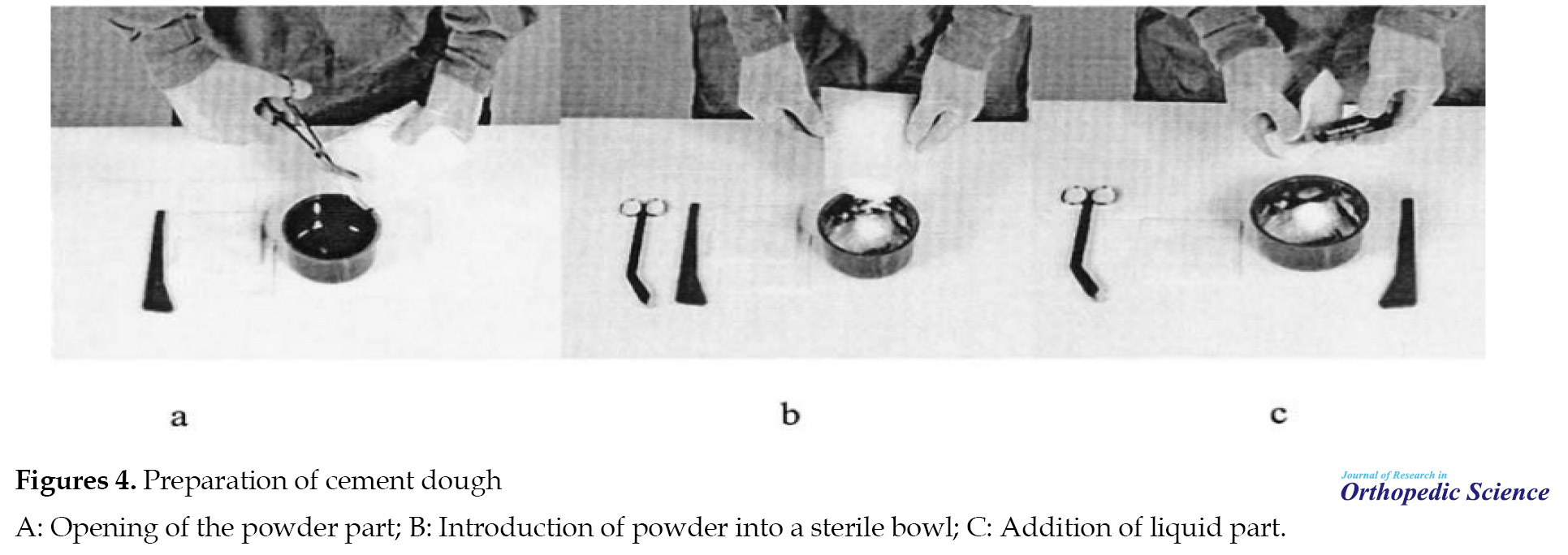
As soon as the liquid and the powder are combined, a chemical reaction occurs in the form of polymerization for PMMA and crystallization for CPC and CSCs, and finally, a solid structure is formed in vivo [
37,
40,
42] which is known as cold curing.
The setting process is classified into four stages: 1) mixing stage 2) waiting stage 3) working stage (manipulation/use of cement), and 4) hardening stage [
31]. Each stage has a different duration that can vary depending on the characteristics of the cement manufacturer [
42,
64,
69,
70,
71]. The steps for making the cement paste are shown in
Figure 5 [
42].
Molecular structure and polymerization reaction
Chemically, upon mixing the two components and performing the reaction between the initiator and activator, primary free radicals are produced, and subsequently, the polymerization process is carried out. Initiation, propagation, and termination are the stages of the free radical polymerization process. The ability of bone cement to perform such a process allows it to self-cure.
Figure 6 depicts the schematics of the reaction mechanism of ABC [
31,
40,
42,
49,
64,
72].
Factors affecting the curing process of acrylic bone cements
ABC brands may be similar or different in characteristics, such as chemical composition, the particle size of prepolymerized PMMA in powder, the particle size distribution of powder, molecular weight of the powder, and cured cement [
49]. The chemical composition of the cement is one of the factors that affect the cement curing process. However, relative humidity, room temperature, thermal changes during cooking [
40,
49,
64], initiator/activator ratio [
40], solid to liquid ratio [
31,
40,
42,
49,
64], changes in radiopacifier [
40], mixing frequency, time of placement in the bone cavity, and the thickness of the cement are the other factors that affect the cement curing process [
64]. Changing the initial chemistry [
40]of PMMA bone cement improves some of the properties of the cement; however, adverse effects on other properties are likely to occur in the process. The polymerization curing parameters of acrylic bone cement which include various characteristics, such as the range and values of paste time, settling time, and peak temperature are fully described in ASTM F451-86 specifications for medical and surgical devices and materials [
64].
Properties required for clinical applications
An IBC is desirable for use in bony defects, particularly in areas with high load bearing when it has the following characteristics [
37]: 1) simple injection and persistent monotony during injection; 2) proper setting time after the combination; 3) slight hazard of necrosis; 4) having suitable mechanical properties (tensile, compressive, and shear strength) according to the characteristics of the desired area, including the amount of load bearing and the amount of movement; 5) resemble firmness to the encompassing bone; 6) excellent, great, supreme, or superb radiopacity (or radiodensity); 7) biological activity; 8) proportionality of the rate of absorption and the rate of tissue formation; 9) adequate micro-porosity (<100 μm) and macro-porosity (more than 100 μm) for the conveyance of nutrient/waste, angiogenesis, cell migration, and high performance of drug delivery within the bone repair process; 10) osteoconductivity and osseointegration. Of all the studies performed, only a few types of cement have shown in vivo performance. IBCs should create a texture around the spongy bone and be able to mold to the shape of the defect during the injection. In addition to not interfering with the healing process, IBCs must be biologically suitable for new bone growth at the implant site. Simple manipulation is also extremely important for the economic fulfillment of alternatives to injectable bone grafting [
5,
20,
31,
39,
64].
The mentioned parameters are also applied to the clinical applications of calcium phosphate bone cement [
34].
Drawbacks of polymethyl methacrylate bone cements
PMMA cement was first used as a bone substitute and had a positive incentive for progression in artificial joints [
5]. However, there are some potential problems and risks that need to be addressed: 1) exothermic reaction during polymerization reaction (varies from 80°C to 124°C) [
33,
34,
40,
49]; 2) the presence of residual monomer and leakage of monomer without reaction into the surrounding tissues [
31,
34,
37,
40,
42,
49]; 3) it has been found that radiolucent bone cement has decreased the mechanical strength of PMMA and CPC; 4) porosity is widely discussed in bone cement fractures [
40,
73]; 5) shrinkage of cement during sintering of bone cement [
40]; 6) sterilization, in turn, has a detrimental effect on fatigue performance and cement failure resistance [
40]; 7) insufficient mechanical and biological properties [
31,
37,
40,
41,
42,
49,
74].
Improving the ingredients of bone cement
By investigating some of the causes of breakdown in PMMA bone cement, the focus is on techniques that can fix the problems. Therefore, there is a constant interest and need to develop a new generation of bone cement to overcome the limitations of current commercial cement. Over the past 25 years, much research was carried out on bone cement. It is specified that PMMA with various additives gives the mixture a set of physical, chemical, and biological properties [
33,
42,
49,
62,
76]. Nanotechnology can also be used to augment the characteristic of bone cement. Given that the number of studies conducted in this field is limited, so much advancement can be expected [
31,
75]. The increasing popularity and desirability of nanomaterials for the development of cement are because natural bones (such as human thigh bones) are composites made up of nanometer components [
37,
40,
41]. It is necessary to mention that any change or modification in a specific feature or parameter should not negatively influence other properties and adding any substance to improve some of the features should be done with consideration of other features [
34] (
Figure S1). In recent years, methods for synthesizing, functionalizing, and manufacturing additives have greatly evolved, but more research is ongoing to develop clinical applications and reduce the side effects of using them.
Additives to reduce the processing temperature
The search to overcome the problem of high temperatures and adverse biological effects led to the design and manufacturing of a new formulation of bone cement called Boneloc (polymethyl methacrylate / n-decyl methacrylate / isobronyl methacrylate). Other studies have also shown that the addition of nanomaterial MgO particles (average diameter less than 50 nm) to PMMA powder increases the thermal conductivity (consequently, the reaction with less exotherm) [
40,
49].
Bioactive osteogenic agents
Various biologically active factors, such as bone morphogenic proteins or growth factors (growth hormone, beta growth factor, and insulin growth factor) [
34,
76]; rhTGF-81 factor [
34], bone marrow collagen [
42]; ionic additives, such as calcium (Ca2+), silicon (Si2+), magnesium (Mg2+) and strontium (Sr2+) [
37,
40,
42,
46,
76,
77], and titanium dioxide (TiO2) [
31,
40,
46] can improve biocompatibility, ossification properties, coherence, proliferation, and differentiation of inherently biological inert substances. Recently, the consequences of the research have proven that the mixture of nanoparticles, including alumina and titania SiO2, MgO, BaSO4, and ZrO2. Meanwhile, the surfaces of biomaterials have enhanced cellular activity compared to the surfaces with microparticles [
37].
Hydroxyapatite (HA) [
31,
33,
42,
49,
63,
73], foams containing self-adjusting gelatin HA, injectable cement made from HA deficient calcium, and foamed gelatin [
37,
42] as well as nHA in biological IBCs, either independently or mineralized on CNTs or collagen or other materials have been proposed to treat bone defects and have been shown to promote bone growth [
37].
Calcium phosphates [
34,
37,
49,
76] and their enhanced forms are obtained by adding various ions, such as Si, Mg, Sr [
5,
46], and polycations, such as poly (ethyleneimine) and poly (allylamine hydrochloride [
49]. In addition, some compounds, including proteins, polysaccharides (chitosan) [
46,
78], Salt-Hydroxypropyl Methylcellulose (Si-HPMC), Silk Fibrin (SF) [
46], fibers (fiber C and glass phosphate), or polymers (polylactic acid [PLA]) [
37,
42,
46,
79] have been used in bone repair.
Recent research has shown that silicate-based bioceramics (such as akermanite) possess excellent mechanical properties, such as osteoinductivity, and osteogenic and angiogenic differentiation in vitro (such as a variety of stem cells, bone marrow stromal cells, adipose-derived stem cells, periodontal ligament, and human aortic endothelial cells) [
80]. Other types of bioactive ceramics, such as bone cement containing apatite and wollastonite (AWC) [
41,
49], cement containing tri-ethylene-glycol-dimethacrylate (TEGDMA), and PMMA/silica nanocomposite [
33] have been used.
Various features, such as remarkable biological compatibility, bioactivity, and osteoinductivity have made bioactive glasses (BG) to be considered one of the most encouraging synthetic materials for bone repair [
37,
41,
42,
43,
49]. Different types of bioactive glass and their composites (such as 45S5, Ceravital, A / W glass ceramics, polyethylene-bioactive glass mixtures, polysulfone-bioactive glass, and polyethylene-hydroxyapatite Hopex [
11,
33,
41,
43,
49] are used for orthopedic or dental applications.
Antimicrobial additives
Microbial contamination after bone cement implantation, especially after joint arthroplasty surgery is one of the considerable concerns of surgeons [
37,
74]. In an attempt to solve these problems, antimicrobial agents or antibiotics (such as gentamicin, penicillin, erythromycin, vancomycin, temocillin, tobramycin, cefuroxime, colistin) [
34,
40,
42,
49,
74,
76,
81], bacteriocins [
62], as well as nanoparticles in the form of nanospheres, nanosheets, nanofibers or nanotubes, [
74,
82] including silver nanoparticles (n-Ags) [
37,
76], gold nanoparticles [
62,
74], chitosan nanoparticles [
37], PMMA nanocomposites containing 2D MgP nanofibers, and 1D HA nanofibers [
74] have been used as additives in the bone cement mixture.
Additives for self-healing
In nature, there are self-healing substances that can restore their shape and function in part or whole after re-injury. Spontaneous repair of skin tears or repair of broken bones are examples of such substances. Recently, there has been extensive research into the production (manufacturing) and development of self-healing materials. During the last 10 years, plenty of studies have been performed on numerous self-healing polymeric biomaterials and hydrogels for clinical applications. In addition, various materials, such as encapsulated 2-Octyl Cyanoacrylate (OCA), and apatite cement with C-fiber reinforcement have been added as additives to the current bone cement composition [
76,
79].
Additives for radiopacity
As mentioned earlier, adding radiopaque materials may have disadvantages [
49,
64]. A new generation of acrylic bone cement with mechanical properties and long-term clinical performance is required. For this purpose, various studies have been conducted on the synthesis of reinforced cement with various additives [
29]. Organic compounds of bismuth, such as bismuth triphenyl [
30,
37,
64], Bismuth Salicylate (BS) [
40], alumina particles [
30], x-ray opaque iodine-containing 2- (2, 3,5-tri-iodo-benzoyl) ethyl methacrylate (TIBMA), 3,5-dihydrin salicylic methacrylate (DISMA), 2- (4-Iodobenzyl) -oxo-ethyl-methacrylate (4-IEMA), 2, 5-dihydro-8-quinolyl methacrylate (IHQM), Iodo-Hexol (IHX), and iodine xanol (IDX), in the liquid part of bone cement have been investigated [
30,
64]. In addition, other biocompatible nanoparticles [
31,
37,
40,
41], such as iron oxide, alumina [
37], strontium-modified titanium nanotubes [
41], and tantalum pentoxide [
49] have been proven to enhance IBC radiopacity.
Improving mechanical performance
Researchers have made many efforts to improve mechanical properties and have used various additives for this purpose. For instance, a small amount of graphite fiber [
49], aramid fiber and carbon [
31,
49], Ultra-High Molecular Weight Polyethylene Fibers (UHMWPE) [
31,
49], or PMMA fibers [
49], metal alloys stainless steel and vitallium in the form of wires, 316L stainless steel, short fibers, zirconia fibers (ZrO2) [
31], Kelvar fiber -29, Bone particles titanium [
31,
49], stainless steel ferrous compounds (magnetite) [
41,
42] (new ferrous biphasic porous apatite cements modified with iron/alpha tricalcium phosphate [IM / α-TCP]), and calcium sulfate dihydrate (CSD) (IM / α-TCP / CSD-BC) [
50], biopolymers, such as chitosan, cellulose, collagen, and so on [
34,
42,
76,
78] to the acrylic cement matrix have been reported. The use of nanoparticles and nanofibers is another way to improve the mechanical properties of IBCs [
37]; for instance, titanium nanofibers and nanotubes [
31,
63], nano-sized titanium particles [
31], Other nanotubes [
31,
40,
41,
76], organically Modified Montmorillonite (MMT) nanoparticles [
31,
37], calcium carbonate nanoparticles (Colacryl B866) [
37], micro and nano alumina fillers [
41,
74].
Novel formulations
Multi-material cement
As previously mentioned, the use of single materials faces problems. Therefore, various multi-material cement has recently been designed with the characteristic feature of combining the benefits of different materials. Cortoss™ Cerament™ from Bone Support AB, KyphOsFS™, and ActivOs™ from Medtronic are good examples of such composite cement with enhanced properties. Some of the properties of Cortoss are 1) low viscosity 2) the use of a non-vaporizable liquid monomer which after combination turns into a paste with the consistency of toothpaste and maintains this state until it polymerizes (quickly within a few seconds), 3) the slight exothermic temperature within the polymerization process (setting reaction) (~63 °C), 4) module near the spongy bone, 5) good biological activity, and 6) ability to create a cement-bone interface which is reinforced during the time, and the bone can be placed on that surface without any fibrous interference [
83].
Drug-loaded bone cement
CPCs as bioactive substances [
42] include gene or ion transport carriers (e.g., calcium, phosphate, strontium, silicate, zinc, and magnesium); various proteins (bone morphogenetic protein family [BMP]); growth factors, such as vascular endothelial growth factor (VEGF); peptides platelet-rich plasma (PRP), bone marrow autologous concentrate (BMC) [
5,
34,
40,
42,
45,
46,
82, 84]; and drugs, such as bisphosphonates for the treatment of osteoporosis [
34,
42,
45,
46], antibiotics [
45], anticancer drugs (DOXO) and agents to control osteomyelitis [
41, 85], analgesics (bupivacaine or ropivacaine, contrast agents [
46], radiopaque agent Xenetix [
42,
46], plasmids or small interfering RNAs (siRNAs) RNAs [
46]. nHA is also considered a drug carrier for alendronate (hydrophilic drug) and can also improve drug encapsulation efficiency in injected PCL microspheres [
37].
Gel-Based injectable materials with in-situ polymeric transverse connection
The injection of gel-based materials is another new system designed for in vivo freezing. For example, the relatively new materials are polyethylene glycol (PEG), polycaprolactone (PCL), PEG, poly (lactic-co-glycolic acid), PEG, polyvinyl alcohol-acrylamide, and alginate-based on in situ polymer gels. These materials were originally used in controlled drug delivery, but have other bone and cartilage repair applications. Also, another function of these gels is the efficient transfer of nutrients and waste during the bone regeneration process, which is due to the special structure of these gels that act as porous channels in situ [
37].
2. Conclusion
Although many years have passed since the discovery of these cement, a supreme IBC that fulfills all clinical needs has not been created, and there are still many issues to consider. Hence, bone cement research has focused on improving the quality and eliminating or reducing the undesirable side effects of bone cement. In this regard, efforts are made to modify them and achieve their desirable properties by adding various materials, including common acrylate polymers, fibers, antibiotics, mineral components such as HA, bioactive glasses, rare elements, biological agents, and nanostructured materials. Various studies have shown that there is a very delicate balance in changing chemical composition. Therefore, researchers should be careful to check all the properties before any application, because increasing any additive may cause change (increase/decrease) in other properties. As a result, this study was conducted to investigate new techniques to help prevent the failure of cemented joint replacement. Recent advances in technology in the development of vertebroplasty or balloon kyphoplasty methods rely on creative ideas and have highlighted the need for interdisciplinary collaboration. Advances in this area have provided opportunities to improve biomaterials with more sophisticated functions and use new approaches. In addition, if achieved, these improvements will not only reduce the burden of health care services but also improve the patient and improve the quality of life after surgery. Given these issues, there is great potential for further development, and complete alternative materials are still on the way. A very promising future can be imagined.
Recommendations for clinical research
- The use of other new additives and their adjustments with a more detailed examination of the properties according to each of the challenges mentioned to improve the properties of bone cement.
- In vivo studies of small animals can be performed to understand better biocompatibility, mechanical strength, and ossification potential, followed by controlled clinical trials in humans to demonstrate clinical efficacy. For a definite conclusion, research based on similar objective parameters is needed.
-Long-term follow-up of bone cement in human participants.
Ethical Considerations
Compliance with ethical guidelines
There were no ethical considerations to be considered in this research.
Funding
This research did not receive any specific grant from funding agencies in the public, commercial, or not-for-profit sectors.
Conflict of interest
The authors declared no conflict of interest.
References
- Henkel J, Woodruff MA, Epari DR, Steck R, Glatt V, Dickinson IC, et al. Bone regeneration based on tissue engineering conceptions - A 21st-century perspective. Bone Res. 2013; 1(3):216-48. [DOI:10.4248/BR201303002] [PMID] [PMCID]
- Zimmermann EA, Busse B, Ritchie RO. The fracture mechanics of human bone: Influence of disease and treatment. Bonekey Rep. 2015; 4:743-55 [DOI:10.1038/bonekey.2015.112] [PMID] [PMCID]
- Meyers MA, Chen PY, Lin AY, Seki Y. biological materials: Structure and mechanical properties. Prog Mater Sci. 2008; 53(1):1-206. [DOI:10.1016/j.pmatsci.2007.05.002]
- Wegst UGK, Bai H, Saiz E, Tomsia AP, Ritchie RO. Bioinspired structural materials. Nat Mater. 2015; 14(1):23-36. [DOI:10.1038/nmat4089] [PMID]
- Liu C, He H. Developments and applications of calcium phosphate bone cementsss. Singapore: Springer; 2018. [Link]
- Heini P, Franz T, Fankhauser C, Gasser B, Ganz R. Femoroplasty augmentation of mechanical properties in the osteoporotic proximal femur: A biomechanical investigation of PMMA reinforcement in cadaver bones. Clin Biomech. 2004; 19(5):506-12. [DOI:10.1016/j.clinbiomech.2004.01.014] [PMID]
- WHO. Assessment of fracture risk and its application to screening for postmenopausal osteoporosis: Report of a WHO study group [meeting held in Rome from 22 to 25 June 1992]. Geneva: World Health Organization; 1994. [Link]
- Cummings SR, Melton LJ. Epidemiology and outcomes of osteoporotic fractures. Lancet. 2002; 359(9319):1761-67. [DOI:10.1016/S0140-6736(02)08657-9]
- Arealis G, Nikolaou VS. Bone printing: New frontiers in the treatment of bone defects. Injury. 2015; 46(8):S20-2. [DOI:10.1016/S0020-1383(15)30050-4]
- Cai Z, Wan Y, Becker ML, Long YZ, Dean D. Poly (propylene fumarate)-based materials: Synthesis, functionalization, properties, device fabrication, and biomedical applications. Biomaterials. 2019; 208:45-71. [DOI:10.1016/j.biomaterials.2019.03.038] [PMID]
- Duan H, Cao C, Wang X, Tao J, Li C, Xin H, et al. Magnesiumalloy rods reinforced bioglass bone cements composite scaffolds with cortical bonematching mechanical properties and excellent osteoconductivity for loadbearing bone in vivo regeneration. Sci Rep. 2020; 10(1):18193-207. [DOI:10.1038/s41598-020-75328-7] [PMID] [PMCID]
- Agarwal R, Garcia AJ. Biomaterial strategies for engineering implants for enhanced osseointegration and bone repair. Adv Drug Deliv Rev. 2015; 94:53-62. [DOI:10.1016/j.addr.2015.03.013] [PMID] [PMCID]
- Feng P, Wu P, Gao C, Yang Y, Guo W, Yang W, et al. A Multimaterial scaffold with tunable properties: Toward bone tissue repair. Adv Sci. 2018; 5(6):1700817. [DOI:10.1002/advs.201700817] [PMID] [PMCID]
- Kim JA, Yun HS, Choi YA, Kim JE, Choi SY, Kwon TG, et al. Magnesium phosphate ceramics incorporating a novel indene compound promote osteoblast differentiation in vitro and bone regeneration in vivo. Biomaterials. 2018; 157:51-61. [DOI:10.1016/j.biomaterials.2017.11.032] [PMID]
- Yang B, Yin J, Chen Y, Pan S, Yao H, Gao Y, et al. 2D-black-phosphorus-reinforced 3D-printed scaffolds: A stepwise countermeasure for osteosarcoma. Adv Mater. 2018; 30(10). [DOI:10.1002/adma.201705611] [PMID]
- Long M, Rack HJ. Titanium alloys in total joint replacement-a materials science perspective. Biomaterials. 1998; 19(18):1621-39. [DOI:10.1016/S0142-9612(97)00146-4]
- Diba M, Tapia F, Boccaccini AR, Strobel LA. Magnesium-containing bioactive glasses for biomedical applications. Int J Appl Glass Sci. 2012; 3(3):221-53. [DOI:10.1111/j.2041-1294.2012.00095.x]
- Jahan K, Tabrizian M. Composite biopolymers for bone regeneration enhancement in bony defects. Biomater Sci. 2016; 4(1):25-39. [DOI:10.1039/C5BM00163C] [PMID]
- Nandi SK, Roy S, Mukherjee P, Kundu B, De D. K, Basu D. Orthopedic applications of bone graft & graft substitutes: A review. Indian J Med Res. 2010; 132:15-30. [Link]
- Bohner M. Resorbable biomaterials as bone graft substitutes. Mater Today. 2010; 13(1-2):24-30. [DOI:10.1016/S1369-7021(10)70014-6]
- DiMaio FR. The science of bone cement: a historical review. Orthopedics. 2002; 25(12):1399-407. https://journals.healio.com/doi/abs/10.3928/0147-7447-20021201-21
- Vert M, Doi Y, Hellwich K-H, Hess M, Hodge P, Kubisa P, et al, Terminology for biorelated polymers and applications (IUPAC Recommendations 2012). Pure Appl Chem. 2012; 84(2):377-410. [DOI:10.1351/PAC-REC-10-12-04]
- Lewis G. Viscoelastic properties of injectable bone cements for orthopedic applications: State-of-the-art review. J Biomed Mater Res B Appl Biomater. 2011; 98(1):171-91. [DOI:10.1002/jbm.b.31835] [PMID]
- Shi H, Zhang W, Liu X, Zeng S, Yu T, Zhou C. Synergistic effects of citric acid - Sodium alginate on physicochemical properties of α-tricalcium phosphate bone blocks of cement. Ceram Int. 2019; 45(2 Part A):2146-52. [DOI:10.1016/j.ceramint.2018.10.124]
- Staden ADV, Dicks LMT. Calcium orthophosphate-based bone cements (CPCs): Applications, antibiotic release, and alternatives to antibiotics. J Appl Biomater Funct Mater. 2012; 10(1):2-11 [DOI:10.5301/JABFM.2012.9279] [PMID]
- Larsson S, Bauer TW. Use of injectable calcium phosphate cement for fracture fixation: A review. Clin Orthop Rel Res. 2002; (395):23-32. [DOI:10.1097/00003086-200202000-00004] [PMID]
- Nottrott M, Mølster AO, Moldestad IO, Walsh WR, Gjerdet NR. Performance of bone cements: Are current preclinical specifications adequate? Acta Orthop. 2008; 79(6):826-31. [DOI:10.1080/17453670810016920] [PMID]
- Kowalski R, Schmaehling R. Commercial aspects and delivery systems of bone cements. In: Deb S, editor. Orthopedic bone cements. Boca Raton: CRC; 2008. [DOI:10.1533/9781845695170.1.113]
- Shah M. The clinical outcome of bone cements in dental implant insertion- A systematic review. J Dent Implant. 2020; 10(2):59-71. [DOI:10.4103/jdi.jdi_11_20]
- Khaled SMZ. Development of a new generation of bone cements using nanotechnology (Spine title: Nanocomposite bone cements) [PhD, Dissertation]. London: University of Western Ontario; 2009. [Link]
- Rentería-Zamarrón D, Cortés-Hernández DA, Bretado-Aragón L, Ortega-Lara W. Mechanical properties and apatite-forming ability of PMMA bone cements. Mater Des. 2009, 30(8):3318-24. [DOI:10.1016/j.matdes.2008.11.024]
- Rabiee SM. [New generation of bone cements (Persian)]. J Babol Univ Med Sci. 2010; 12(2):53-61. [Link]
- Klammert U, Reuther T, Blank M, Reske I, Barralet JE, Grover LM, et al. Phase composition, mechanical performance and in vitro biocompatibility of hydraulic setting Calcium Magnesium Phosphate cement. Acta Biomater. 2010; 6(4):1529-35. [DOI:10.1016/j.actbio.2009.10.021] [PMID]
- Ostrowski N, Roy A, Kumta PN. Magnesium phosphate cement systems for hard tissue applications: A review. ACS Biomater Sci Eng. 2016; 2(7):1067-83. [DOI:10.1021/acsbiomaterials.6b00056] [PMID]
- No YJ, Roohani-Esfahani SI, Zreiqat H. Nanomaterials: The next step in injectable bone cementsss. Nanomedicine (Lond). 2014; 9(11):1745-64. [DOI:10.2217/nnm.14.109] [PMID]
- Morejón-Alonso L, Carrodeguas RG, dos Santos LA. Development and characterization of α-tricalcium phosphate/monocalcium aluminate composite bone cements. J Biomed Eng. 2012; 5(8):448-56. [DOI:10.4236/jbise.2012.58057]
- Bardají S, Borrós S. New developments in vertebroplasty materials. AFINIDAD. 2018; 75(583):165-74. [Link]
- Ayre WN. Novel approaches to the development of PMMA bone cements [PhD, Dissertation]. United Kingdom: Cardiff University; 2013. [Link]
- Liu-Snyder P, Webster TJ. Developing a new generation of bone cements with nanotechnology. Curr Nanosci. 2008; 4(1):111-8. [DOI:10.2174/157341308783591799]
- Yousefi AM. A review of Calcium Phosphate cements and acrylic bone cements as injectable materials for bone repair and implant fixation, J Appl Biomater Funct Mater. 2019; 17(4):2280800019872594. [DOI:10.1177/2280800019872594] [PMID]
- Zhu T, Ren H, Li A, Liu B, Cui C, Dong Y, et al. Novel bioactive glass-based injectable bone cements with improved osteoinductivity and its in vivo evaluation. Sci Rep. 2017; 7(1):3622-31. [DOI:10.1038/s41598-017-03207-9] [PMID] [PMCID]
- Thomas MV, Puleo DA. Calcium sulfate: Properties and clinical applications. J Biomed Mater Res B Appl Biomater. 2009; 88(2):597-610. [DOI:10.1002/jbm.b.31269] [PMID]
- Hemmati K, Hesaraki S, Nemati A. Evaluation of ascorbic acid-loaded calcium phosphate bone cements: Physical properties and in vitro release behavior. Ceram Int. 2014; 40(3):3961-68. [DOI:10.1016/j.ceramint.2013.08.042]
- He Z, Zhai Q, Hu M, Cao C, Wang J, Yang H, et al. Bone Cements for percutaneous vertebroplasty and balloon kyphoplasty: Current status and future developments. J Orthop Translat. 2015; 3(1):1-11 [DOI:10.1016/j.jot.2014.11.002] [PMID] [PMCID]
- Gauthier O, Bouler JM, Weiss P, Bosco J, Daculsi G, Aguado E. Kinetic study of bone ingrowth and ceramic resorption associated with the implantation of different injectable Calcium-Phosphate bone substitutes. J Biomed Mater Res. 1999; 47(1):28-35. [DOI:10.1002/(SICI)1097-4636(199910)47:13.0.CO;2-P]
- Barrack RL, Mulroy RDJ, Harris WH. Improved cementing techniques and femoral component loosening in young patients with hip arthroplasty. A 12-year radiographic review. J Bone Joint Surg Br. 1992; 74:385-89. [DOI:10.1302/0301-620X.74B3.1587883] [PMID]
- Serbetci K, Hasirci N. Recent developments in bone cements. In: Yaszemski MJ, editor. Biomaterials in orthopedics. 2th ed. New York: CRC Press; 2003. [DOI:10.1201/9780203913086.ch12]
- Vlad MD, Valle LJ, Poeata I, Lopez J, Torres R, Barraco M, et al. Biphasic Calcium Sulfate Dihydrate/iron-modified alpha-tricalcium phosphate bone cements for spinal applications: In vitro study. Biomed Mater. 2010; 5(2):25006-18 [DOI:10.1088/1748-6041/5/2/025006] [PMID]
- Yang G, Liu J, Li F, Pan Z, Ni X, Shen Y, et al. Bioactive Calcium Sulfate/ Magnesium Phosphate cement for bone substitute applications. Mater Sci Eng C Mater Biol Appl. 2014; 35:70-6. [DOI:10.1016/j.msec.2013.10.016] [PMID]
- Schmalz G, Bindslev DA. Biocompatibility of dental materials. Berlin: Springer; 2009. [Link]
- Dionysopoulos D; Tolidis K; Tortopidis D; Gerasimou P; Sfeikos T. Effect of a Calcium Chloride solution treatment on physical and mechanical properties of glass ionomer cements. Odontology. 2018; 106(4):429-38. [DOI:10.1007/s10266-018-0338-5] [PMID]
- Mickenautsch S, Yengopal V, Banerjee A. Retention of orthodontic brackets bonded with resin-modified GIC versus composite resin adhesives-a quantitative systematic review of clinical trials. Clin Oral Investig. 2012; 16(1):1-14. [DOI:10.1007/s00784-011-0626-8] [PMID]
- ISO 9917-1:2007 - Dentistry - Water-based cements - Part 1: Powder/liquid acid-base cements. 2th ed. 2007. [Link]
- Baig MS, Fleming GJP. Conventional glass-ionomer materials: A review of the developments in glass powder, polyacid liquid and the strategies of reinforcement. J Dent. 2015; 43(8):897-912. [DOI:10.1016/j.jdent.2015.04.004] [PMID]
- Sidhu SK. Glass-ionomers in dentistry. London: Springer; 2015. [DOI:10.1007/978-3-319-22626-2]
- Moshaverinia A, Ansari S, Mohajerani M, Roohpour N, Darr JA, Rehman I. Effects of incorporation of hydroxyapatite and fluoroapatite nanobioceramics into conventional glass ionomer cements (GIC). Acta Biomater. 2008; 4(2):432-40. [DOI:10.1016/j.actbio.2007.07.011] [PMID]
- Zainuddin N, Karpukhina N, Law RV, Hill RG. Characterisation of a remineralising Glass Carbomer® ionomer cement by MAS-NMR spectroscopy. Dent Mater. 2012; 28(10):1051-8. [DOI:10.1016/j.dental.2012.06.011] [PMID]
- Lautenschlager EP, Stupp SI, Keller JC. Structure and properties of acrylic bone cements in the book: In: Ducheyne P, Hastings GW, editors. Functional behavior of orthopaedic biomaterials: Applications. Florida: CRC Press; 1984. [Link]
- Harper EJ, Bonfield W. Tensile characteristics of ten commercial acrylic bone cements. J Biomed Mater Res. 2000; 53(5):605-16. [DOI:10.1002/1097-4636(200009)53:5<605::AID-JBM22>3.0.CO;2-5]
- Vaishya R, Chauhan M, Vaish A. Bone cements. J Clin Orthop Trauma. 2013; 4(4):157-63. [DOI:10.1016/j.jcot.2013.11.005] [PMID] [PMCID]
- Khaled SZ, Rizkalla AS, Charpentier PA. Development of a new generation of bone cements using nanotechnology [PhD. dissertation]. Ontario: University of Western Ontario; 2009. [Link]
- Vázquez B, Abraham G.A, Elvira C, Gallardo A, San Román J. Key properties and recent advances in bone cements technology. Dordrecht: Springer; 2002. [Link]
- Lewis G. Effect of methylene blue on the fracture toughness of acrylic bone cement. Biomaterials. 1994; 15(12):1024-8. [DOI:10.1016/0142-9612(94)90085-X]
- Lohbauer U. Dental glass ionomer cements as permanent filling materials?—Properties, limitations future trends. Materials. 2009; 3(1):76-96. [DOI:10.3390/ma3010076]
- Nicholson JW. Adhesion of glass-ionomer cements to teeth: A review. Int J Adhes Adhes. 2016; 69:33-8. [DOI:10.1016/j.ijadhadh.2016.03.012]
- Nicholson JW, Brookman PJ, Lacy OM, Wilson AD. Fourier transform infrared spectroscopic study of the role of tartaric acid in glass-ionomer dental cements. J Dent Res. 1988; 67(12):1451-4. [DOI:10.1177/00220345880670120201] [PMID]
- Havelin LI, Espehaug B, Vollset SE, EngesAeter LB. The effect of the type of cement on early revision of Charnley total hip prostheses. A review of eight thousand five hundred and seventy-nine primary arthroplasties from the Norwegian Arthroplasty Register. J Bone Joint Surg Am. 1995; 77(10):1543-50. [DOI:10.2106/00004623-199510000-00009] [PMID]
- Babaie E, Lin B, Goel VK, Bhaduri SB. Evaluation of amorphous magnesium phosphate (AMP) based non-exothermic orthopedic cements. Biomed Mater. 2016; 11(5):055010. [DOI:10.1088/1748-6041/11/5/055010] [PMID]
- Race A, Miller MA, Mann KA. A modified PMMA cement (Sub-cement) for accelerated fatigue testing of cemented implant constructs using cadaveric bone. J Biomech. 2008; 41(14):3017-23. [DOI:10.1016/j.jbiomech.2008.07.016] [PMID] [PMCID]
- Krause M, Soltau M, Zimmermann EA, Hahn M, Kornet J, Hapfelmeier A, et al. Effects of long-term alendronate treatment on bone mineralisation, resorption parameters and biomechanics of single human vertebral trabeculae. Eur Cell Mater. 2014; 28:152-63. [DOI:10.22203/eCM.v028a12] [PMID]
- Phakatkar AH, Shirdar MR, Qi ML, Taheri MM, Narayanan S, Foroozan T, et al. Novel PMMA bone cement nanocomposites containing magnesium phosphate nanosheets and hydroxyapatite nanofibers. Mater Sci Eng C Mater Biol Appl. 2020; 109:110497. [DOI:10.1016/j.msec.2019.110497] [PMID]
- Paz E, Forriol F, del Real JC, Dunne N. Graphene oxide versus graphene for optimisation of PMMA bone cement for orthopaedic applications. Mater Sci Eng C Mater Biol Appl. 2017; 77:1003-11. [DOI:10.1016/j.msec.2017.03.269] [PMID]
- Totaro NP. Recent advances and additives of bone cements and bone augments for arthroplastic surgeries [MSc, Thesis]. United States: Louisiana State University; 2015. [Link]
- Li Z, Yuan N, Lam RW, Cui Z, Yang X, Lu WW. Preclinical evaluation of strontium-containing bioactive bone cement. Mater Sci Eng C Mater Biol Appl. 2013; 33(8):5100-4. [DOI:10.1016/j.msec.2013.07.017] [PMID]
- Fang CH, Lin YW, Sun JS, Lin FH. The chitosan/tri-calcium phosphate bio-composite bone cement promotes better osteo-integration: An in vitro and in vivo study. J Orthop Surg Res. 2019; 14(1):162. [DOI:10.1186/s13018-019-1201-2] [PMID] [PMCID]
- Boehm AV, Meininger S, Gbureck U, Müller FA. Self-healing capacity of fiber-reinforced Calcium Phosphate cements. Sci Rep. 2020; 10(1):1-3. [DOI:10.1038/s41598-020-66207-2] [PMID] [PMCID]
- Chen L, Zhai D, Huan Z, Ma N, Zhu H, Wu C, et al. Silicate bioceramic/PMMA composite bone cement with distinctive physicochemical and bioactive properties. RSC Adv. 2015; 5(47):37314-22. [DOI:10.1039/C5RA04646G]
- Xu YM, Peng HM, Feng B, Weng XS. Progress of antibiotic-loaded bone cement in joint arthroplasty. Chin Med J (Engl). 2020; 133(20):2486-94. [DOI:10.1097/CM9.0000000000001093] [PMID] [PMCID]
- Amiri S, Yeganeh A, Moghtadaei M. Simvastatin and ezetimibe-loaded nanofibers administered locally to augment bone healing. J Orthop Spine Trauma. 2020; 6(3):75-6. [DOI:10.18502/jost.v6i3.4972]
- Lewis G. Injectable bone cements for use in vertebroplasty and kyphoplasty: State-of-the-art review. J Biomed Mater Res B Appl Biomater. 2006; 76(2):456-68. [DOI:10.1002/jbm.b.30398] [PMID]
- Bagherifard A, Jabalameli M, Jahansouz A, Yahyazadeh H, Karimi Heris H, Khezri M, et al. Platelet-rich plasma injection for symptomatic knee osteoarthritis. J Res Orthop Sci. 2015; 2(3). [DOI:10.17795/soj-2022]
- Dewhurst RM, Scalzone A, Buckley J, Mattu C, Rankin KS, Gentile P, et al. development of natural-based bone cements for a controlled doxorubicin-drug release. Front Bioeng Biotechnol. 2020; 8:754. [DOI:10.3389/fbioe.2020.00754] [PMID] [PMCID]